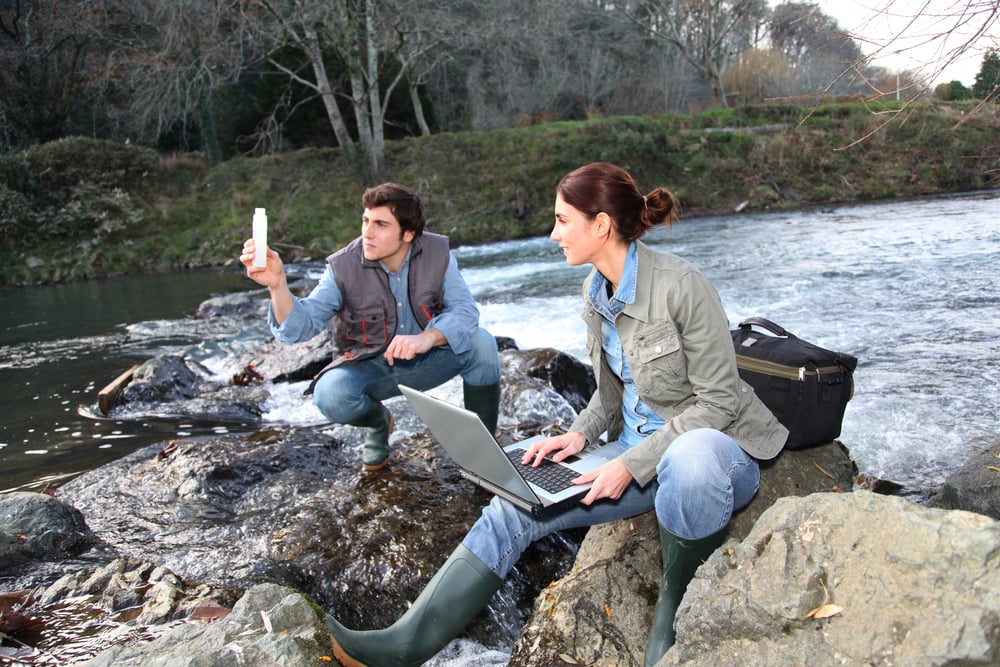
This article is the second of a two part series to highlight how carbon nanotubes can be used to filter and purify our most vital resources. In part 1 we covered air purification and common methods used to provide clean air. In this second part we discuss drinkable water and the ongoing challenge of filtration and purification that comes with making that resource pure and safe to consume.
Hundreds of millions of people around the world lack access to drinkable water, or even water that has been purified to any acceptable standard. Providing sustainable access to clean water in areas that are still developing is a critical challenge. Many methods of water purification and filtration exist today but not all are energy efficient, not all of them can be scaled properly for all levels of usage, and some are difficult or impossible to apply in certain areas.
One might think that since water covers over 70% of the world's surface it would be easy to obtain plentiful drinkable water; however, saltwater is not safe to drink, and the salt is difficult to remove. Even a large body of freshwater typically holds many toxins and harmful particles, requiring that the water be circulated through some kind of treatment or cleaning station. Various filtration methods to remove toxins and desalination processes that can remove salt from saltwater must be used in conjunction to provide the world with a steady supply of drinkable water. Of course, no single option is designed to combat every hazard, and there is a need to further develop these methods to improve efficiency and widen the range of toxins that can be dealt with.
Water Filters
Standard water filter options utilize common materials such as carbon or sand with a range of pore sizes and shapes. Filters are designed to have internal surface areas with many microscopic pores that can trap particles and toxins as water passes through the filter. As with air filters, small pores are crucial for trapping the smallest particles, but small pores also clog quickly as they trap material, reducing the usable life of the filter and limiting flow; this can be offset by using a larger surface area, extending the usage time before the filter needs to be replaced.
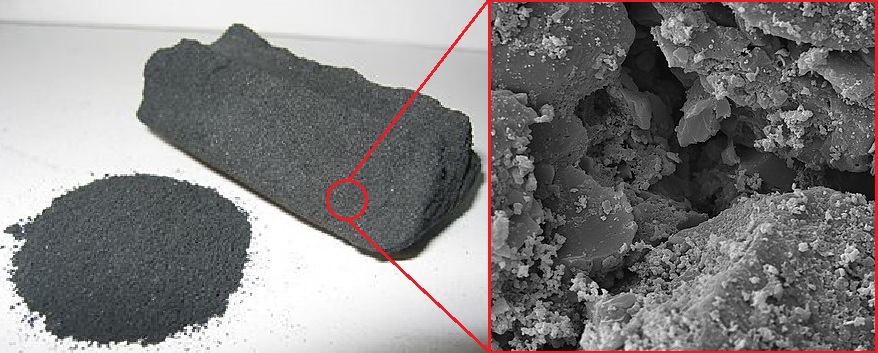
One common type of water filter is an activated carbon water filter. Activated Carbon filters are designed to effectively capture particles down to 0.5 µm in size. They can be manufactured using any carbonaceous materials like coal, coconut shells, and wood; these materials are exposed to hot gases to open microscopic pores in the material, thus activating it for use. This process is what gives the carbon a very large surface area and a pore structure that maximizes its ability to catch particulates. The image above shows a pile of activated carbon granules alongside a close-up image of the pore structure. Just one pound of activated carbon can contain hundreds of acres of surface area for trapping toxins. One of the benefits of these filters is that they are generally inexpensive to create and can be optimized for different applications (size, flow rate, increased lifespan, etc.) which aids in maximizing efficiency of water filtration.
Activated carbon filters can be paired with other types of filters to aid in targeting other particles in the water. An activated carbon filter is most effective in removing odors, sediments, chlorine, and other organic compounds (VOC’s). For particularly challenging water sources that are rich with minerals, salt, and other inorganic substances, a second filter process may be more appropriate, such as filtration through greensand, which is a sandy rock sediment.
Unfortunately, about 97% of our water available is saltwater or brackish water, which cannot easily be cleaned by most conventional filters due to the extremely small size of salt ions. To make use of this resource, we need another purification method.
Water Desalination (Reverse Osmosis)
Why are there so many people without access to drinkable water if most of them live near a body of water already? The answer is that turning saltwater or brackish water into drinkable water is a very energy-intensive process, and unfortunately many communities in developing regions that lack access to clean drinking water also lack the sufficient amount of electric power needed to run the process. Simple filtration of fresh water may be more feasible, but streams and ponds may be few and far between compared to the large available bodies of salt and brackish water. For the sake of these developing communities, and to ensure that drinking water can remain available and affordable for everyone in the world in the future, it is critical to achieve more efficient or low-energy desalination technology.
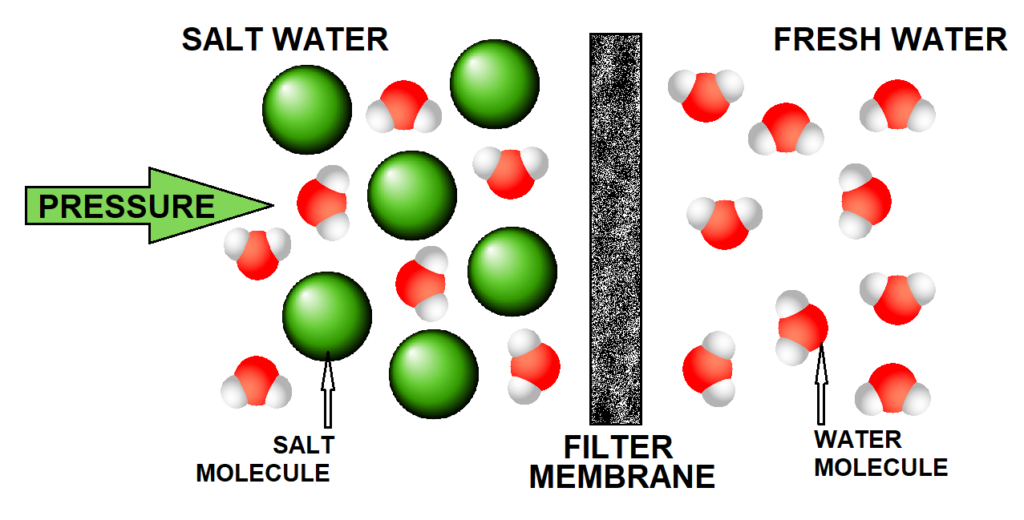
The image above is a simple representation of reverse osmosis, a process currently used to desalinate water. The process becomes more complicated and varied when carried out at larger scale, but as a simple scenario one can imagine a tank of saltwater on one side of the membrane connected to a high pressure pump; this high pressure forces water through a filter membrane that traps the salt molecules, building up a store of pure water located on the other side. This process relies on the membrane having pore size large enough to allow the pure water molecules through but small enough to trap the salt molecules.
Although reverse osmosis is much more energy efficient than alternative desalination methods such as distillation (which requires significant heat energy), it can still require a great deal of energy, depending on the starting salt content of the water. Thus, desalination is still restricted to small scale uses in areas in which energy is relatively cheap, and filtration of fresh water using conventional filtration is only an option where natural fresh water sources are plentiful. A better solution is needed.
Carbon Nanotubes
Carbon nanotubes (CNTs) have a combination of properties that allow them to fit into both of the broad application areas mentioned above. CNTs can be extremely small, with some types measuring 0.001 - 0.002 µm in diameter; their cylindrical structure and strong carbon-carbon bonds make them relatively stiff and durable, which allows them to form stable overlapping networks with extremely high porosity and surface area, perfect for mechanical filtration. This construction is suitable for water filters of both types previously discussed.
CNTs are superior to activated carbon in that they can potentially remove all types of pollutants, including minerals, salt, inorganic substances, organic substances, and microorganisms. Aside from physically stopping pollutants and toxins during a filtration process, CNTs also have antimicrobial properties which aid in producing safe drinking water.
The ability of CNTs to effectively filter E.coli out of water has been demonstrated by researchers in the Department of Chemical Engineering at Yale University. These researchers have worked with both single wall carbon nanotubes (SWCNTs) and multi-wall carbon nanotubes (MWCNTs) with a variety of diameters and lengths, and have studied their respective antimicrobial effects. Their studies, which can be found here and here and which are discussed in a portion of this review article, have shown that both SWCNTs and MWCNTs excel at destroying bacteria and microorganisms even after short exposure times. The diameter, length, structure, and number of walls of the CNTs are all important factors in determining their antimicrobial properties. CNTs with smaller diameters and greater lengths showed a higher antibacterial performance when agglomerated in liquids; this is attributed to such CNTs forming aggregates with higher surface areas available for bacterial contact, indicating that a solid filter of networked CNTs may have a similar effect.
Because of the unique shape of CNT molecules, overlapping them to form porous networks is not the only way to build them into a filter: they can also clean water by allowing water molecules to pass down the hollow centers of the cylindrical molecules, rejecting particles that are larger than the CNT diameter. A filter composed of well-aligned CNTs with very small diameters can be a good replacement for the membrane filters currently used in reverse osmosis systems. Ironically, the fact that CNTs are hydrophobic (slightly repellent to water molecules), makes it possible to pass water through them with very little energy; this effect was described here in 2006. Water molecules within in center of a CNT molecule will tend to be repelled from the walls and corralled into the center of the hollow tube; for the thinnest CNTs, with diameters of 0.8 - 1.0 nanometers, this means that water molecules are induced to flow through the CNT core in a single file line, without much friction or adhesion with either the molecular walls or other water molecules. With a slight pressure gradient to drive the water into the CNTs in the first place, this can create a high-throughput filtration without the need for significant energy, while still excluding other molecules.
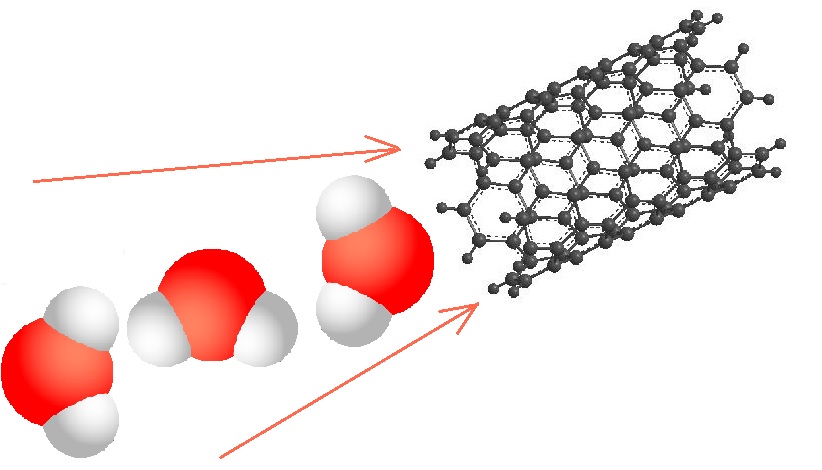
The hydrogen bonds between water molecules promote this process, making it stable and repeatable. When the correct diameter of CNTs is used, the hydrophobic channels induce water to break into single-file chains, and entropic effects cause these chains to spontaneously flow to the other end of the channel, yielding pure water on the far side. The ability of CNTs to form aligned bundles with extremely high surface area and a large number of channels allows this filtration technique to be highly effective. This type of filtration has already been tried commercially; Porifera is an example of a company that has patented filter membranes that use this technique.
Conclusion
Water purification, and water desalination in particular, are technologies that will be critical to society in the coming decades, and CNT filter membranes may be a key to improving technology in this area. It is possible that DexMat’s method for producing aligned carbon nanotube fibers and films could one day be modified to produce a structure with high porosity and an extremely large effective surface area. Ideally, a technology that can align and arrange CNTs into a structure that aids in the filtration or desalination of water at lower energy consumption will allow engineers of the near future to bring drinkable water to those who face the greatest challenges in obtaining it.